Last Updated on: 24th May 2025, 03:22 pm
As ports around the world push forward on their decarbonization journeys, the final and perhaps most challenging frontier is decarbonizing the vessels themselves—not only within the harbor but throughout their voyages. This fourth and culminating phase of port electrification and decarbonization strategy tackles precisely this challenge, extending the benefits of clean electrification far beyond port boundaries and into the very propulsion systems powering maritime trade.
This logical progression builds upon the successful groundwork established in the initial five years, when ground vehicles were electrified, the focus of the second five years, electrifying port vessels and ferries, and then the third five years, when cold ironing of major ships is introduced. The baseline energy demand was established in the introductory article. This particular order is simplified to allow a particular part of port energy demands to be assessed. In reality, ground vehicles, port, inland and short sea vessels and shore power will be electrifying with fits and starts somewhat in parallel, with ground vehicles ahead, and vessels and shore power likely occurring in parallel.
By the 2040s, the technology landscape for maritime electrification will have significantly evolved. Battery energy densities, charging infrastructures, and renewable generation capabilities will have improved dramatically, making previously ambitious scenarios commonplace. The result: a maritime sector poised to operate largely or entirely without fossil fuels within coastal and inland routes. Inland shipping, already an efficient and environmentally favorable mode of freight transportation, represents an ideal candidate for full electrification. By this stage, inland barges are anticipated to operate predominantly on battery-electric propulsion systems, supported by modular battery-swapping stations located strategically at major ports. These battery modules, designed to standardized dimensions similar to shipping containers, would be rapidly swapped out at ports, ensuring minimal disruption to shipping schedules and maximizing operational flexibility.
In parallel, short-sea shipping routes—those coastal voyages often spanning distances of just a few hundred kilometers—would similarly be transformed through electrification. Vessels on these routes, including feeder container ships of up to around 2,000 TEU and coastal Ro-Pax ferries, are ideally suited to battery-electric solutions due to their predictable, short-range operations, although the biggest ships on the longest routes might still be hybrid electric. High-power shore-side charging systems installed during earlier phases at major ports as well as containerized battery swapping would now enable these short-sea vessels to quickly recharge at each port call, fully eliminating onboard combustion during voyages. A strong proof point for this are the two 700 TEU container ships plying 1,000 km routes on the Yangtze in China, swapping depleted containerized batteries for charged ones at ports along the route.
For ocean-going vessels that cover vast distances, the energy demands remain significantly higher, making full electrification challenging even by mid-century. Yet substantial electrification is achievable, particularly within designated coastal emission control areas extending approximately 200 kilometers offshore. Within these zones, large ships would switch seamlessly to onboard battery power, sailing silently and emissions-free into and out of port. This hybrid propulsion approach dramatically reduces local emissions, significantly improving coastal air quality and aligning shipping operations with stringent regulatory standards. Upon arriving in port, these ocean-going vessels would connect to high-capacity shore power systems already installed in prior electrification phases or once again take advantage of swappable containerized batteries, fully recharging their batteries during typical berth times. On departure, they would continue on battery power until beyond the coastal emissions zone, at which point they could switch to renewable biofuels or synthetic fuels for deep-ocean segments of their journeys.
Biofuels play an essential complementary role in decarbonizing long-haul maritime transportation. Recognizing that battery capacity, even with dramatic improvements, remains impractical for multi-thousand-kilometer ocean voyages, sustainable drop-in fuels such as hydrotreated vegetable oils (HVO) or biomethanol become necessary. By this final phase, ports will no longer supply traditional fossil-based bunker fuels. Instead, port bunkering infrastructure transitions fully to bio-derived fuels, ensuring even the longest ocean crossings maintain carbon-neutral propulsion. Vessels receiving fuel at these ports would thus carry renewable energy supplies sufficient for their entire oceanic journey, dramatically reducing global maritime emissions and positioning these ports as hubs for fully sustainable shipping practices.
As a note, I’m bullish on biodiesel as opposed to biomethanol simply because it can be blended with current VLSFO in increasing percentages over a decade or two in existing bunkering facilities, and provides the same energy density as VLSFO, two substantial advantages over biomethanol. However, others such as Paul Martin, are more bullish on biomethanol because its feedstocks are much simpler and more available, so they expect the more limited higher quality feedstocks to be preserved for sustainable aviation biokerosene. My projections of demand for aviation and shipping, as well as my assessments of biofuel feedstocks and processes suggest that demand will be much lower than current projections and feedstocks are far more than adequate, but it’s an open point at present.
There is strong potential for ships which shuttle back and forth across the Atlantic to be fully electric in the future. A study out of Berkeley Lab in 2022 found that it wasn’t mass or volume that was a constraint, but battery costs. While the study was imperfect, it found that at $100 per kWh, 1,500 routes were economically breakeven without subsidies, and 3,000 km routes were economic at $50 per kWh. We’re already seeing $60 range full battery pack prices for LFP out of China’s grid storage auctions, and we’re likely to see that trend down well below $50 with chemistry, manufacturing and pack innovations in the coming years. 3,000 km is the distance between Ireland and Newfoundland, so while this energy projection doesn’t include journeys of that distance, it’s very much within the realm of the possible. At minimum, the 200 km on either end of the journey currently projected will likely extend as far as possible due to the cost advantages of cheap electrons vs more expensive fuels, with operators optimizing energy as much as possible.

As a note on this, my projections of maritime shipping decarbonization exclude synthetic fuels for the simple reason that they will always be more expensive than biofuels. Hydrogen can be green but it can’t be cheap, something I and a few others have been pointing out for years, and now everyone else is realizing as organizations do what should have been done from the beginning, robust technoeconomic modeling with realistic assumptions.
A few key fallacies underpin the myth of green hydrogen being cheap. The first is that complex chemical processing plants with 28 mostly commoditized components would experience price reductions like more novel and massively manufactured items like batteries and solar panels, which are only starting to reach the end of the s-curves of price reductions. The second is that these expensive and complex electrolysis plants could operate 30% of the time on otherwise curtailed and hence very cheap electricity, ignoring the impact of amortizing capital costs across the reduced output. The third is that hydrogen would be effectively free to distribute, ignoring the high costs of storage, transmission and distribution of hydrogen, as well as the high costs of synthesizing molecules from scratch.
Unfortunately, in the late 2010s major and credible organizations such as the BNEF, IEA, CSIRO, LUT and PIK all gave the job of projecting hydrogen costs to people incompetent to do the work, and they all came up with deeply unrealistic cost projections. A contact tells me the IEA assigned the job to an intern. As far as I can tell, they figured out what price point green hydrogen would have to be at in order to be economically realistic as an energy carrier, then bent the laws of physics and economics to justify that cost point, instead of putting up their hands and admitting it didn’t make sense. Joe Romm, who worked at the DOE in the 1990s funding hydrogen initiatives, did the math while working on the first edition of his book, “The Hype About Hydrogen” over 20 years ago and realized the reality of the situation and said so.
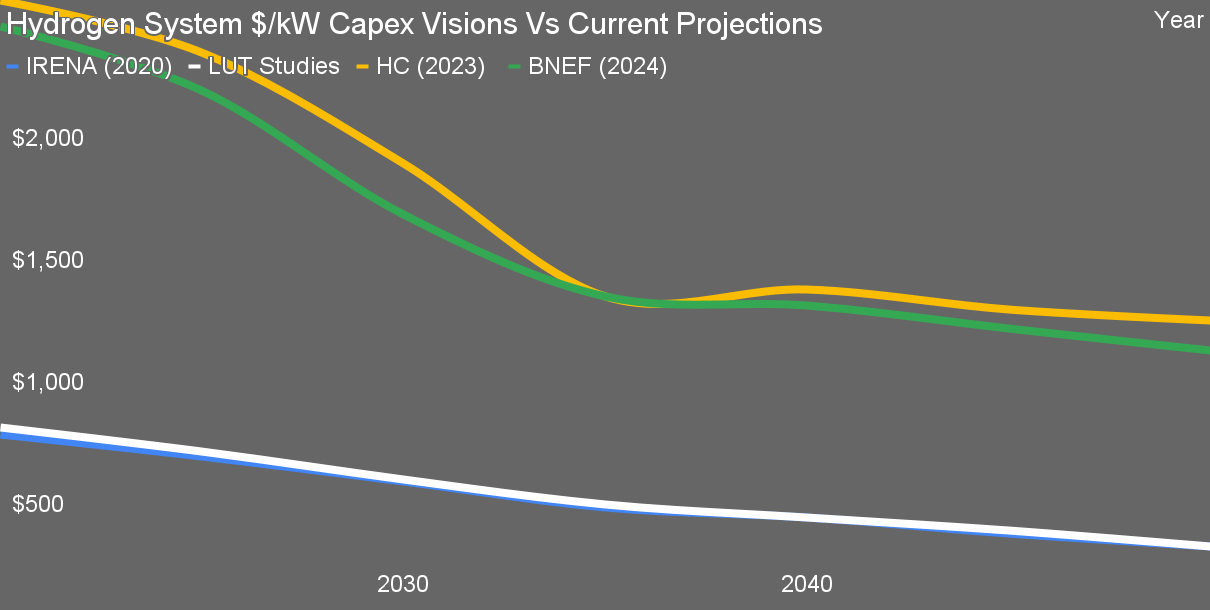
The organizations have been slowly increasing their cost projections each year since 2020, but only BNEF has had the courage to admit it was completely wrong, tripling its cost projections for electrolysers for 2050 recently. As a result, green hydrogen cost projections are still anchored far too low in most policy makers and strategists minds, leading to an ongoing delusion that synthetic fuels will pencil out.
Returning to port electrification, the transition of inland, short-sea, and partially hybridized blue-water vessels to electric propulsion naturally leads to a substantial increase in electricity demand. Inland barges and short-sea vessels alone would collectively draw tens of gigawatt-hours of electricity per year. The hybridized propulsion systems on large ocean-going vessels, each potentially requiring tens of megawatt-hours per visit to recharge their sizable batteries, further escalate electricity demands. By mid-century, total annual port electricity consumption would be around 80 GWh—roughly five times greater than the initial baseline at the outset of the decarbonization journey.

Meeting this unprecedented electrical demand necessitates significant expansions in renewable generation, particularly offshore wind. By the mid-2040s, the port would aim to secure upwards of 100 megawatts of offshore wind capacity, comfortably generating approximately 350 gigawatt-hours annually at typical offshore wind capacity factors. This ample renewable generation not only covers the port’s extensive electricity needs but also provides substantial surplus power, which could either feed back into national grids or be used to operate electrified chemical processing plants and biofuel refineries, creating additional revenue opportunities and reinforcing energy security. Solar power installations, potentially augmented by large-scale offshore solar platforms, would further contribute to a robust, diverse renewable energy portfolio. The proof point for the platform-based offshore solar is once again China, where cities already have GW-scale offshore solar farms on seabed mounted platforms.
Energy storage and management emerge as critical enabling technologies in this final phase. The massive influx of electricity demand at peak charging times, especially when multiple large vessels simultaneously require battery recharging, necessitates robust, large-scale battery storage solutions. By this stage, ports are expected to deploy battery energy storage capacities on the order of 200 to 300 megawatt-hours, capable of delivering rapid, high-power discharges—on the scale of tens of megawatts—to manage intense charging periods. Such storage systems also play key roles in smoothing intermittent renewable generation, capturing excess wind and solar production during low-demand periods and discharging during demand spikes. Advanced grid management techniques, smart charging scheduling, and potentially even vehicle-to-grid solutions adapted to ships (using docked vessel batteries as dynamic energy resources) further enhance system stability, ensuring reliable and resilient power supply.

It’s worth contextualizing the energy demands of ports compared to blue water shipping. Early in the series I noted that a version with the liquid fuels still required would be very lopsided, with shipping fuels dwarfing all other energy flows. In the end state, it’s even worse as port and short sea shipping energy requirements drop radically with electrification, while biofuels will only have the efficiency of bunker fuels today. While we won’t need nearly as much maritime shipping fuel as most projections assert, ignoring as they do the plummeting of bulks and electrification, we’ll still need 70 million tons annually in my projection. As IEA shows, however, we already make 100 million tons of biofuels annually, with 70 million tons being biodiesel, we’re just wasting it on ground transportation, for the most part.
The investments required to realize this ambitious vision are significant, estimated in the range of several hundred million euros. Expanded offshore wind generation alone would likely require around €200 million, while large-scale battery storage infrastructure would add tens of millions more. High-power charging stations, extensive battery-swapping facilities, and upgraded biofuel bunkering infrastructure similarly contribute substantial capital requirements.
Despite the high capital costs, the economic rationale remains compelling. Rapidly declining costs of batteries and renewables, combined with rising fossil fuel costs due to carbon pricing and tightening regulations, create a robust financial case. Furthermore, such infrastructure investments are likely supported by substantial European and national governmental incentives, reflecting broad public and policy recognition of maritime decarbonization’s critical importance. Major ports cost billions of euros to build or expand substantially and have annual revenues of a billion euros, so low hundreds of millions aren’t a showstopper in the port space.
The completion of this fourth and final phase represents not merely a strategic infrastructure investment but a transformative leap forward in maritime sustainability and competitiveness. Ports adopting these comprehensive decarbonization measures early position themselves as indispensable hubs in a new era of global shipping—one defined not by fossil fuels, but by renewable energy, electrified propulsion, and deep sustainability.
In the coming world, bulk shipping is going to plummet and container shipping won’t grow to match the losses. 40% of oceanic shipping are of coal, oil and gas, and another 15% are of raw iron ore. All four of those commodities are in structural decline between the rapid growth of renewables, grid batteries and EVs, and China’s infrastructure build out reaching completion, and decarbonized shipping will be more expensive shipping, also changing economics to favor more local processing. In the coming world, we’ll have too much of the wrong kind of port capacity, pure bulk ports will be trying desperately to become container ports, existing container ports will have a strong advantage and electrified ports will have the biggest advantage. The ports that survive the coming shake out will be the ones that read the tea leaves early.
Maersk is an example of this, with its APM Terminals divisions’ 75 or so concessions for major container ports around the world electrifying ground operations under the guidance of Sahar Rashidbeigi, someone I’ll finally be meeting in person while in northeastern Europe assisting Tennet with 2050 scenario planning this summer.
This holistic approach to maritime electrification, addressing emissions from inland barges through to ocean-going vessels, ensures that ports fully achieve their zero-carbon objectives while significantly enhancing their long-term operational resilience and market leadership. As regulatory environments continue to evolve rapidly in response to climate urgency, proactive ports implementing these advanced electrification strategies will undoubtedly lead the maritime sector into its sustainable future.
Sign up for CleanTechnica’s Weekly Substack for Zach and Scott’s in-depth analyses and high level summaries, sign up for our daily newsletter, and/or follow us on Google News!
Whether you have solar power or not, please complete our latest solar power survey.
Have a tip for CleanTechnica? Want to advertise? Want to suggest a guest for our CleanTech Talk podcast? Contact us here.
Sign up for our daily newsletter for 15 new cleantech stories a day. Or sign up for our weekly one on top stories of the week if daily is too frequent.
CleanTechnica uses affiliate links. See our policy here.
CleanTechnica’s Comment Policy
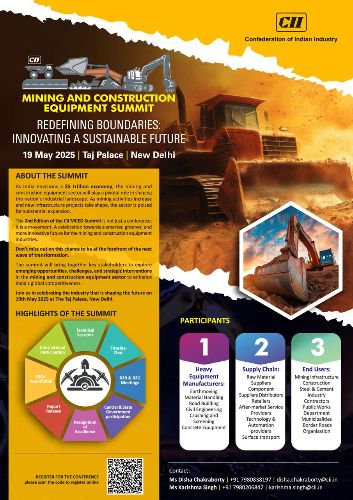